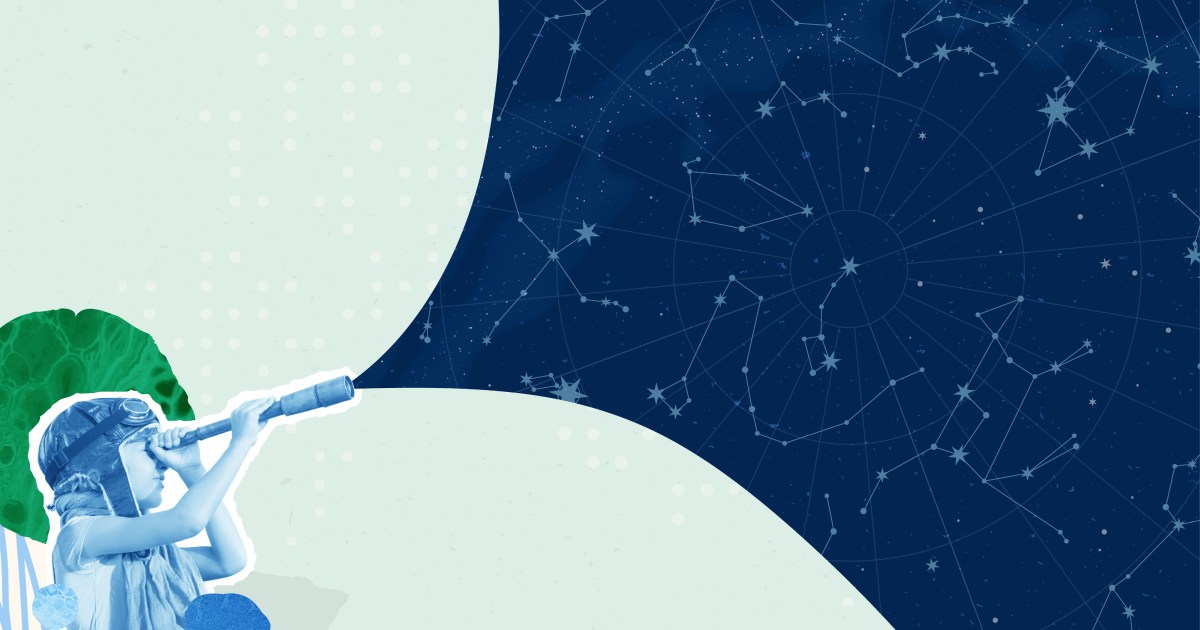
Octant: A Next Generation Approach to Genetic Disease
Octant: A Next Generation Approach to Genetic Disease
As we’ve begun IND-enabling studies on a small-molecule corrector for Rhodopsin-associated autosomal dominant Retinitis Pigmentosa (RHO-adRP), we thought it would be a good time to reflect on our approach to drug discovery, and why we’re excited about it.
Over the last several years, we’ve built a next-generation approach to engineer small molecule correctors for protein misfolding diseases. We now have novel, home-grown chemical matter in multiple drug programs, including the first orally available small molecule to treat RHO-adRP, a serious blinding disease with no standard of care. This breakthrough is not only exciting because it could be life-changing for patients by saving their sight, but also because it validates a unique approach that is repeatable across many of the other diseases caused by protein misfolding.
WHERE ARE ALL THE SMALL MOLECULE CORRECTORS?
There is incredible value to be unlocked in small molecule correctors. These molecules function like small molecule gene therapies, addressing the root cause of disease with less costly molecules that can penetrate most tissues and cell types. Vertex Pharmaceuticals demonstrated this approach against cystic fibrosis, one of the many protein misfolding diseases. It was a stunning achievement for patients. A handful of other therapies, like Pfizer’s Vyndamax (ATTR) and Amicus’ Galafold (Fabry disease), have also validated small molecule correctors, unlocking significant treatments for patients.
Given the success of these therapeutics, why haven’t there been more correctors? Given the large market incentives (Vertex’s Trikafta is a ~$10B/year franchise), where are all the me-too and fast followers one would see for almost any other therapeutic area? They’re not there because engineering correctors is hard, and it requires an approach at odds with how the industry currently discovers and develops drugs.
First, misfolding manifests in emergent cellular processes like folding, proteostasis, post-translational modifications, trafficking, and signaling that can only be studied in human cells. Vertex, the scion of structure-based drug discovery, acquired Aurora Biosciences, a cellular screening company, to get their program working in just one disease. Human cell engineering and screening at scale across multiple genes, variants, and functional outcomes is incredibly complex.
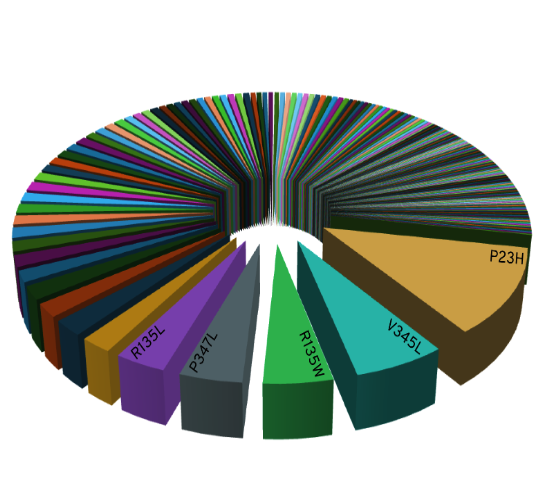
Second, unlike cystic fibrosis and sickle cell disease, which are dominated by a single gene variant, most misfolding diseases are genetically diverse. Most monogenic diseases have hundreds of causal variants. Understanding which variants do what, and whether a single molecule can be engineered to treat enough patients to make a program clinically and commercially feasible has been beyond the reach of traditional approaches.
Finally, most correctors developed to date have been repurposed inhibitors. Early hits in corrector programs have poor drug-like qualities requiring extensive optimization. And because misfolded proteins are difficult to structure or biochemically purify, chemists are left without most of the tools traditionally used to optimize chemical matter.
We’ve built the platform to tackle these obstacles:
CHALLENGE #1: MECHANISTIC COMPLEXITY OF CELLULAR PHENOTYPES
RHO-adRP is caused by mutations to rhodopsin, the critical protein for the rod cells in your eyes. For many patients, the disease is caused by the protein getting stuck in the cell’s protein production pathway, causing severe cellular dysfunction and then cell death. Early loss of low-light vision seriously impacts patients' quality of life, and their peripheral and central vision deteriorates over the course of their lives, leading to eventual blindness.
We used synthetic biology to build human cells engineered with sensors that report out on the many different interactions caused by these misfolded proteins. Individually barcoded cells enable us to interrogate protein function, protein interactions, signaling, abundance, stability, and trafficking. We also use counter-screens to understand whether the compounds we’re screening affect these activities with specificity to that particular protein, or whether we are causing background or off-target effects.
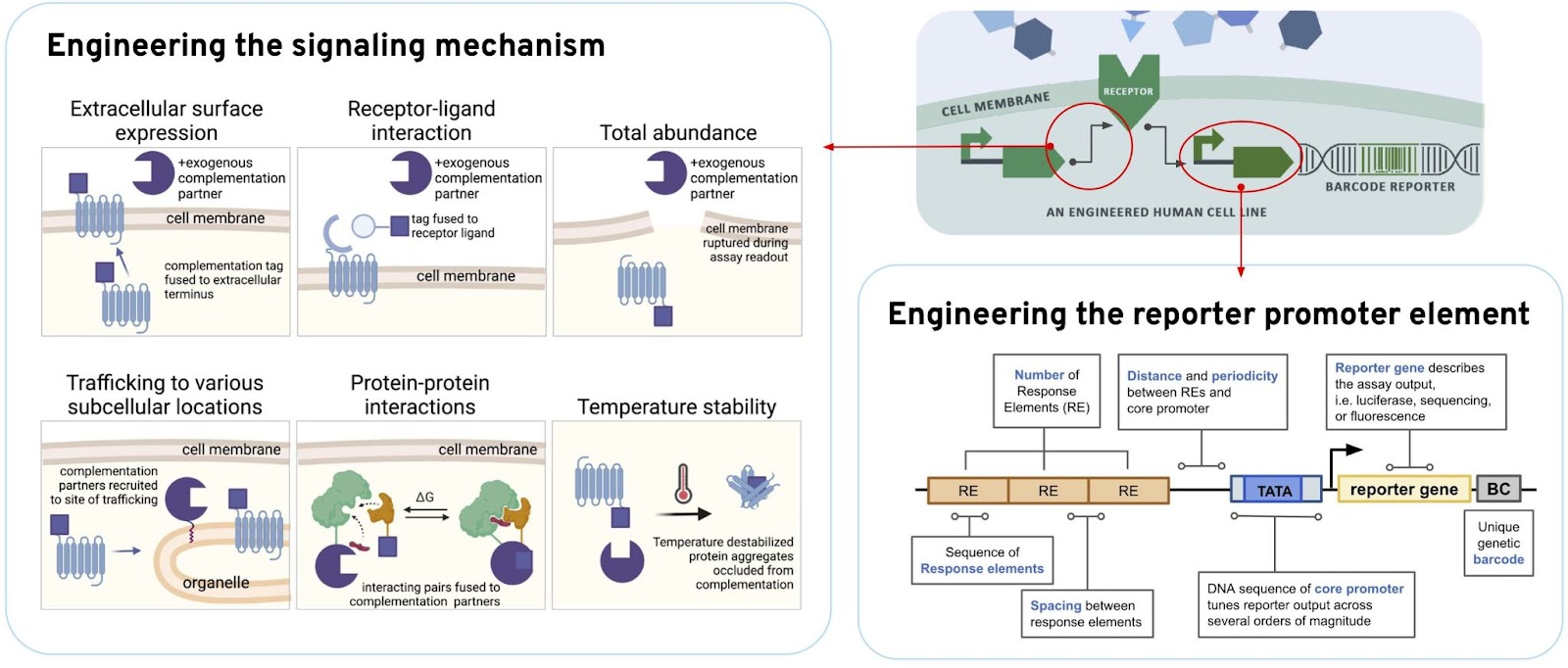
CHALLENGE #2: GENETIC HETEROGENEITY
For any given misfolding disease, there can be thousands of genetic variants that lead to the condition. A threshold question for a drug discovery program is whether we can understand what the different mutations are doing mechanistically, and whether enough of them are treatable with the same molecule for a drug program to be clinically and commercially viable.
We used our deep mutational scanning (DMS) technology to build barcoded human cell models for every possible amino-acid substitution of RHO. The DMS reveals which mutations of RHO cause mistrafficking, and we cross-referenced that with patient data to understand if we could build a pan-mutant chaperone that would treat enough patients. The answer is yes!
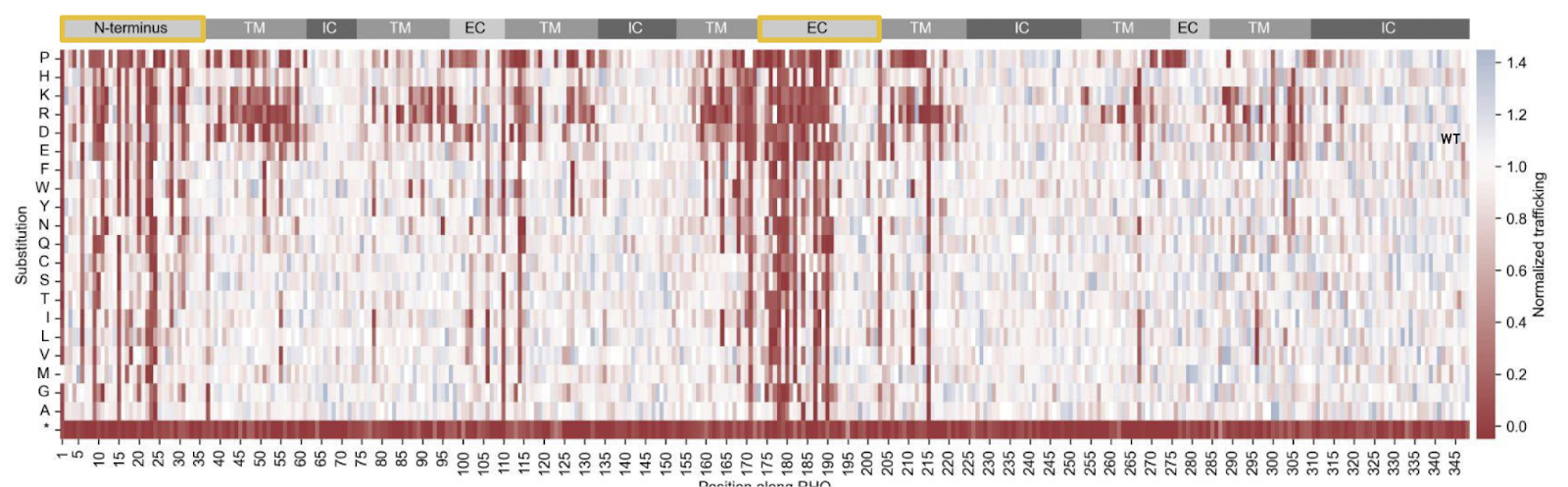
CORRECTOR CHALLENGE #3: POOR STARTING POINTS
We found initial hits that helped traffic rhodopsin, but those molecules were very hydrophobic and had poor drug-like qualities. Extensive optimization was needed to engineer more potent molecules that could get across the blood-retinal barrier and causally treat the disease. We would need to do this without the use of biochemical and structural approaches which typically aid in optimizing molecular properties.
We used our nano-scale chemistry platform to decompose and diversify our molecules, improving their chemical properties. This process iteratively combines different “cores” and “fragments” of our molecules, thousands of combinations at a time, screening them directly into human cells. A lot of the jumps we see in molecular properties are non-additive. The best cores don't always work best with the best fragments. Those rarer non-additive molecules enable significant jumps across scaffolds to help us transform our molecules.
We used this design-build-test-learn cycle, screening 250,000 molecular analogs, 5,000 per week, on a weekly cadence to engineer orally bioavailable molecules that get to the retina with good coverage, selectively engage the target, correct for the disease, and avoid liabilities.

PROOF IN THE PLATFORM PUDDING: THE FIRST CORRECTOR FOR RHO-ADRP
After years of hard work by many Octonauts, we’ve produced novel, high-value chemical matter that we haven’t seen built in any other way.
Take our lead program for RHO-adRP as an example: our corrector therapy is a first-in-class orally bioavailable corrector that crosses the blood-retinal barrier, has great coverage in the retina, and corrects the misfolding of enough variants to cover roughly 50% of RHO-adRP patients.
We’re excited to see that the drug seems to work incredibly well. It helps not only stop degeneration in a P23H mouse model of the disease but also allows regeneration of retina structure and function, all with an orally dosed molecule!

Currently, there is no standard of care for patients living with RHO-adRP. With most therapies focused on ocular injections, this oral small molecule is a new approach that could change the lives of thousands of patients, and we’re working tirelessly to deliver it.
And this is just one program, with many more correctors to come.
NAVIGATING THE FUTURE OF DRUG DISCOVERY AT OCTANT
It’s been a difficult but rewarding journey pushing the boundaries of drug discovery. The Octant platform is a new way of navigating diseases that manifest in the mechanisms of our cells. With each breakthrough, we’re not just pioneering new treatments, we’re also expanding what’s possible in small molecule drug discovery.
Stay tuned as we continue to explore uncharted territories seeking to help patients worldwide.